Dioxins are highly toxic organic compounds that can remain in the environment for extended periods. These persistent organic pollutants (POPs), which include polychlorinated dibenzo-p-dioxins (PCDDs) and polychlorinated dibenzofurans (PCDFs), are mainly generated by the combustion or manufacture of chlorine-containing materials such as plastics. Dioxins and other closely related POPs, such as polychlorinated biphenyls (PCBs), are classed as carcinogenic by the United States Environmental Protection Agency, and present a significant threat to human health even at low levels.
Dioxins and PCBs can enter the food chain when livestock consume contaminated animal feed, and can accumulate in the fatty tissues of animals due to their high fat-solubility. As a result, over 90% of human exposure to dioxins and PCBs is through the consumption of meat, fish, dairy and other foods of animal origin.1 Given the health risks posed by dioxins and PCBs, effective food testing workflows are essential to ensure products do not exceed regulatory-defined safe levels.
GC-MS/MS: A Robust Technique for Analyzing Dioxins and PCBs in Food and Animal Feed
To control human exposure to PCDDs, PCDFs and PCBs from the food chain, global regulatory bodies have established maximum levels (MLs) and action levels (ALs) for various POPs in food products, as well as approved analytical methods for food testing laboratories to enforce these standards. In the European Union (EU), for example, European Commission regulations 2017/644 and 2017/771 outline sampling, sample preparation and analysis protocols for the detection of dioxins and other dioxin-like compounds in food and animal feedstuffs.2,3
With food testing laboratories tasked with handling potentially hundreds of samples every day, these workflows must be supported by robust and reliable analytical technologies that can confidently identify and accurately quantify dioxins and PCBs with minimal maintenance requirements in order to minimize downtime and maximize throughput.
Thanks to ongoing improvements in the robustness and sensitivity of gas chromatography-triple quadrupole mass spectrometry (GC-MS/MS) systems, regulations were updated in 2014 to permit this technique as an alternative to gas chromatography-high resolution mass spectrometry (GC-HRMS) for confirmatory analysis and for the control of MLs and ALs. The latest GC-MS/MS systems are capable of exceptionally reliable performance for the routine analysis of dioxins and PCBs, providing accurate and sensitive quantification of these compounds even at trace levels.
Case Study: Sensitive and Reliable Determination of Dioxins Using GC-MS/MS
The performance of modern GC-MS/MS systems was evaluated in a recent study involving the confirmatory analysis and quantification of 17 PCDDs and PCDFs, and 18 dioxin-like and non-dioxin-like PCBs in solvent standards and various food and feedstuff samples. The samples were analyzed using a triple quadrupole GC-MS/MS system equipped with the advanced electron ionization source (AEI) and a TG-Dioxin capillary GC column. Two identical GC-MS/MS systems in two separate laboratories were used to assess the reproducibility of the method.
Extraction was performed by Twisselmann hot extraction or pressurized liquid extraction. The automated clean-up of the extracts was performed using a three-column setup, comprising multi-layered acidic silica, alumina and carbon columns. Two fractions were generated per sample (the first containing non-ortho PCBs, PCDDs and PCDFs, and the second containing mono-ortho and di-ortho PCBs and indicator PCBs) and these were analyzed separately. The analytical method gave excellent separation of all the PCDD, PCDF and PCB congeners in less than 45 minutes.
Given the high sensitivity of modern GC-MS/MS instruments, a calibration-based approach was used to determine limits of quantitation (LOQs) of the analytical system. Using calibration standards at the LOQ and subsequent check standards at this level enabled the performance of the method to be assessed throughout the analytical sequence. This also allowed LOQs for the individual congeners to be determined, assuming a fixed sample weight. Individual congener LOQs could be applied to upper-bound, middle-bound and lower-bound toxicity equivalence (TEQ) results by substituting the result of any congener that fell below the lowest calibration point with this value multiplied by the toxicity equivalence factor (TEF) of the congener.
To evaluate the response factor deviation over the course of the analytical sequences, standards at the specified LOQ were analyzed at the start, during and end of each run. Using a nominal weight of 2 g, and assuming 100% 13C-labeled standard recovery and all natives were less than the LOQ in the sample, a minimum upper-bound value of 0.152 pg/g WHO-PCDD/F-TEQ was determined. This met regulatory requirements for reporting at 1/5th of the ML upper-bound sum TEQ for all food and feedstuffs with a nominal intake of 2 g, with the exception of guidance associated with liver of terrestrial animals and food for infants or young children, which both have legal limits defined on a fresh weight basis. In these cases, either a larger sample intake or a magnetic sector instrument would be required. All of the calibration sequences demonstrated response factor %RSDs within EU regulations, highlighting the suitability of the method.
To demonstrate the performance of the GC-MS/MS system, six replicate extractions of a mixed fat quality control sample (QK1) were prepared, split between the two sites and analyzed at regular intervals throughout the analytical sequences (14 injections in total). The measured WHO-PCDD/F-TEQ values for congener were in excellent agreement with the reference value provided by the EU Reference Laboratory for Halogenated POPs in Feed and Food, and the upper bound WHO-PCDD/F-TEQ value did not deviate by more than 6% from the reference value for all 14 measurements (Figure 1). The deviation between the upper-bound and lower-bound WHO-PCDD/F-TEQ for each measurement was consistently less than 1.2%, well below the maximum limit of 20% necessary to support compliance with EU regulations.

Robust Routine Analysis of Dioxin and Dioxin-like Compounds
To assess the robustness of the GC-MS/MS system, the PCDD, PCDF and non-ortho PCB extracts were pooled into a mixed matrix sample and analyzed more than 161 injection sequences across a period of approximately two weeks. Each sequence consisted of 40 matrix injections and 40 LOQ standards, interspersed with nonane blanks. No system maintenance, tuning or user intervention was undertaken throughout the two-week study. Figure 2 highlights the exceptional peak area stability achieved for selected PCDD and PCDF congeners.
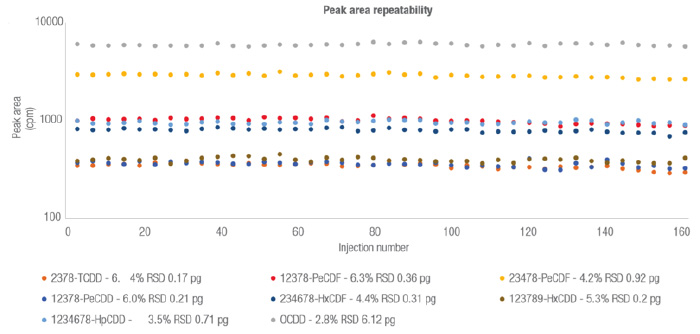
These results highlight the exceptional levels of day-to-day measurement repeatability offered by the latest GC-MS/MS systems. By delivering consistently high performance without the need for extensive maintenance steps, modern GC-MS/MS systems are maximizing instrument uptime and increasing sample throughput for routine POP analysis workflows.
Conclusion
Developments in GC-MS/MS technology, namely the advanced electron ionization source, are pushing the limits of measurement sensitivity, repeatability and robustness to support the needs of routine dioxin and PCBs analysis in food and feed samples. By minimizing instrument downtime while maintaining exceptional levels of analytical performance, these advanced systems are helping high-throughput food testing laboratories to analyze more samples and ultimately better protect consumers from these harmful pollutants.
References
- Malisch, R. and Kotz, A. (2014) Dioxins and PCBs in feed and food – Review from European perspective. Sci Total Environ, 491, 2-10.
- European Commission. Commission Regulation (EU) 2017/644, Off J Eur Union, 2017, L92 9-34.
- European Commission. Commission Regulation (EU) 2017/771, Off J Eur Union, 2017, L115 22-42.
Acknowledgements
This article is based on research by Richard Law and Cristian Cojocariu (Thermo Fisher Scientific, Runcorn, UK), Alexander Schaechtele (EU Reference Laboratory for Halogenated POPs in Feed and Food, Freiburg, Germany), Amit Gujar (Thermo Fisher Scientific, Austin, US), and Jiangtao Xing (Thermo Fisher Scientific, Beijing, China).